LevelDB 源码解析之 Cache 缓存
GitHub: https://github.com/storagezhang
Emai: debugzhang@163.com
LevelDB: https://github.com/google/leveldb
影响数据库读性能的一个重要组件是缓存。如果 LevelDB 的每一次读取都会带来一次磁盘 IO,这些磁盘 IO 就会让系统的整体效率非常低下。
所以,LevelDB 使用了一种基于 LRUCache 的缓存机制,用于缓存:
- 已打开的 sstable 文件对象和相关元数据;
- sstable 中的 dataBlock 的内容。
这种缓存机制使得对热数据的读取尽量在 cache 中命中,避免 IO 调用。
cache 类
Cache
是一个抽象类,声明了一个全局函数:
Cache* NewLRUCache(size_t capacity) { return new ShardedLRUCache(capacity); }
该函数用来创建具有固定容量的缓存,此缓存实现使用最近最少使用的淘汰策略(LRU, least-recently-used),当数据所占内存达到一定阈值时,删掉最近最少使用的数据。
Cache
中的数据是文件的副本,其读取流程为:
- 在 cache 中查找特定的 key,若找到该 key,直接返回对应的 handle,若未找到,执行第 2 步;
- 从文件中读取 key 对应的 entry 到 cache 中,再返回相应的 handle。
接口
Cache
声明了一系列接口:
virtual ~Cache();
- 通过调用
deleter
删除所有的 entry。
struct Handle {};
-
缓存中所存储的项 entry 对应的句柄 handle;
-
除了保存 key-value 数据外还保存了一些维护信息;
-
Handle
是通用接口,此处仅定义空结构体。
virtual Handle* Insert(const Slice& key, void* value, size_t charge,
void (*deleter)(const Slice& key, void* value)) = 0;
- 将一个对应 key-value 的 entry 插入缓存;
- 返回 entry 对应的 handle,当不再需要返回的 handle,调用者必须调用
this->Release(handle)
; - entry 被删除时,key 和 value 将会传递给
deleter
。
virtual Handle* Lookup(const Slice& key) = 0;
- 如果当前缓存中没有对应
key
的 entry,返回nullptr
; - 否则返回 entry 对应的 handle,当不再需要返回的 handle 时,调用者必须调用
this->Release(handle)
。
virtual void Release(Handle* handle) = 0;
- 释放调用
this->Lookup
查找到的 handle:- 对应 entry 的引用计数减 1;
- 若引用计数为 0,调用
deleter
删除该 entry。
- 注意:handle 必须尚未释放。
virtual void* Value(Handle* handle) = 0;
- 返回调用
this->Lookup(key)
查找到的 handle 中封装的值。 - 注意:handle 必须尚未释放。
virtual void Erase(const Slice& key) = 0;
- 从缓存删除
key
对应的 entry。 - 注意:entry 将一直保留,直到 entry 对应的所有 handle 都被
this->Release(handle)
释放。
virtual uint64_t NewId() = 0;
- 返回一个新的数字 id。
- 共享同一缓存的多个客户端可以使用该标识对保存 key 的空间进行分区。
- 通常客户端将在启动时分配一个新的 id,并预先将该 id 添加到它保存 key 的缓存中。
virtual void Prune() {}
- 删除所有不活跃的 entry。
- 内存受限的应用程序可能希望调用此方法以减少内存使用。
virtual size_t TotalCharge() const = 0;
- 返回缓存内存消耗的估计值。
LRUHandle 结构体
LRUHandle
是一个双向循环链表,存储缓存中的 entry,它的功能有:
- 存储 key-value 数据;
- 作为 LRU 链表,是一个可变长度的堆分配结构;
- 记录引用计数并负责清理。
struct LRUHandle {
// 存储的 value 对象的指针,可以存储任意类型的值
void* value;
// 当引用计数 `refs` 降为 0 时的清理函数
void (*deleter)(const Slice&, void* value);
// 哈希表通过链地址法解决哈希冲突,发生哈希冲突时指向下一个 entry
LRUHandle* next_hash;
// LRU 链表双向指针
LRUHandle* next;
LRUHandle* prev;
// 分配的可以消耗的内存量
size_t charge;
// key 的字节数
size_t key_length;
// entry 是否存在缓存中
bool in_cache;
// 引用计数,记录 entry 被不同缓存的引用,用于 entry 删除
uint32_t refs;
// `key()` 对应的哈希,用于快速分区和比较
uint32_t hash;
// 存储 key 的开始地址,结合 `key_length` 获取真正的 key
// GCC 支持 C99 标准,允许定义 char key_data[] 这样的柔性数组(Flexible Array)。
// C++ 标准并不支持柔性数组的实现,这里定义为 key_data[1],这也是 c++ 中的标准做法。
char key_data[1];
Slice key() const {
// 只有当当前节点是空链表头时,`next` 才会等于 `this`
// 链表是循环双向链表,空链表的头节点 next 和 prev 都指向自己构成环
// 链表头是冗余的 handle,不存储数据,只利用它的 next 和 prev
assert(next != this);
return Slice(key_data, key_length);
}
};
HandleTable 类
LevelDB 实现了一个内部哈希表 HandleTable
,它消除了大量的移植漏洞,比一些编译器/运行时组合中的内置哈希表实现更快。例如,随机读的速度比 g++4.4.3
的内置哈希表提高了约 5%。
成员变量
每一个 LRUHandle
即是 HashTable
中的一个哈希桶,相同哈希值的 entry 通过 next_hash
连成链表,同时这些 entry 也通过 next
和 prev
连成为双向链表,最新插入的数据排在链表尾部。
class HandleTable {
public:
HandleTable() : length_(0), elems_(0), list_(nullptr) { Resize(); }
~HandleTable() { delete[] list_; }
private:
// list 数组的长度,即哈希桶的个数
uint32_t length_;
// 存储的元素总个数,当 elems > length 时,扩展 list 数组并重新 hash
uint32_t elems_;
// 存储 entry 的数组,即哈希桶,初始大小为 4,动态扩展,成倍增长
LRUHandle** list_;
}
};
Resize
Resize
函数用于元素增加时动态扩展数组:
void Resize() {
// 初始化时,哈希桶的个数为 4
uint32_t new_length = 4;
// 随着不同哈希值的增加动态扩展,每次扩展为原容量的两倍
while (new_length < elems_) {
new_length *= 2;
}
// 按照新容量申请内存,分配给新链表,并将原数据复制过去
LRUHandle** new_list = new LRUHandle*[new_length];
memset(new_list, 0, sizeof(new_list[0]) * new_length);
uint32_t count = 0;
for (uint32_t i = 0; i < length_; i++) {
LRUHandle* h = list_[i];
while (h != nullptr) {
LRUHandle* next = h->next_hash;
uint32_t hash = h->hash;
LRUHandle** ptr = &new_list[hash & (new_length - 1)];
h->next_hash = *ptr;
*ptr = h;
h = next;
count++;
}
}
assert(elems_ == count);
// 释放原数组
delete[] list_;
// 更新哈希表数据
list_ = new_list;
length_ = new_length;
}
LevelDB 只在 entry 增长的时候扩大 list_
,没有缩小,这符合 LevelDB 的使用场景。
FindPointer
FindPointer
函数返回适合所传入的 key
和 hash
的插入位置:
LRUHandle** FindPointer(const Slice& key, uint32_t hash) {
// list_[hash & (length_ - 1)] 中存储着 next_hash 的地址
// ptr 等于 next_hash 的地址
// *ptr 等于其指向的 next_hash,即指向 next_hash 所连成的链表
// **ptr 等于 next_hash 所指向的 LRUHandle,即指向具体的 entry
LRUHandle** ptr = &list_[hash & (length_ - 1)];
// 利用 *ptr 遍历这个链表,直到找到 key 对应的 entry
// 如果没有找到,ptr 就指向链表的尾部,这最后一个 next_hash 的值是 nullptr
while (*ptr != nullptr && ((*ptr)->hash != hash || key != (*ptr)->key())) {
ptr = &(*ptr)->next_hash;
}
// 返回符合条件的 next_hash 的地址
return ptr;
}
Lookup,Insert 和 Remove
有了 FindPointer
返回的指针,查找函数 Lookup
直接读取指针指向的值:
LRUHandle* Lookup(const Slice& key, uint32_t hash) {
return *FindPointer(key, hash);
}
插入函数 Insert
直接在指针所指向的位置上插入:
LRUHandle* Insert(LRUHandle* h) {
LRUHandle** ptr = FindPointer(h->key(), h->hash);
LRUHandle* old = *ptr;
h->next_hash = (old == nullptr ? nullptr : old->next_hash);
*ptr = h;
if (old == nullptr) {
++elems_;
// 当存储元素个数大于哈希桶个数时,扩展哈希桶数组
// 因为每个缓存项都相当大,所以我们的目标是使链表的平均长度较小(<=1)。
if (elems_ > length_) {
Resize();
}
}
return old;
}
删除函数 Remove
直接删除指针所指向的 next_hash
所指向的 entry:
LRUHandle* Remove(const Slice& key, uint32_t hash) {
LRUHandle** ptr = FindPointer(key, hash);
LRUHandle* result = *ptr;
if (result != nullptr) {
*ptr = result->next_hash;
--elems_;
}
return result;
}
LRUCache 类
LRUCache
是 LRUCache 的内部实现,不对外暴露接口,作为 ShardedLRUCache
的分片存在。
成员变量
class LRUCache {
private:
// 缓存的容量,在初始化时指定
size_t capacity_;
// 互斥锁,保护下列数据
mutable port::Mutex mutex_;
// 缓存中所有 entry 的 charge 总和
// usage 必须小于 capacity
size_t usage_ GUARDED_BY(mutex_);
// 存储 entry 的循环双向链表,链表头冗余,同时维护使用热度
// lru.next 指向最旧的 entry,lru.prev 指向最新的 entry
// 当缓存满了时,先从 lru.next 开始淘汰 entry
// lru 保存 refs==1,并且 in_cache==true 的 entry
LRUHandle lru_ GUARDED_BY(mutex_);
// 存储正在使用的 entry 的循环双向链表,链表头冗余
// 这些 entry 不能被淘汰。
LRUHandle in_use_ GUARDED_BY(mutex_);
// 保存所有 entry 的哈希表,用于快速查找数据
HandleTable table_ GUARDED_BY(mutex_);
};
构造函数和析构函数
LRUCache::LRUCache() : capacity_(0), usage_(0) {
// lru 和 in_use 都是循环双向链表
// 空链表的头节点 next 和 prev 都指向自己构成环,链表头冗余
lru_.next = &lru_;
lru_.prev = &lru_;
in_use_.next = &in_use_;
in_use_.prev = &in_use_;
}
LRUCache::~LRUCache() {
// in_use 链表不能为空
assert(in_use_.next == &in_use_);
for (LRUHandle* e = lru_.next; e != &lru_;) {
LRUHandle* next = e->next;
// entry 必须在缓存中
assert(e->in_cache);
// 将 entry 移除缓存
e->in_cache = false;
// entry 的引用计数必须为 1
assert(e->refs == 1);
// 删除 entry
Unref(e);
e = next;
}
}
Cache 相关接口实现
Lookup
Cache::Handle* LRUCache::Lookup(const Slice& key, uint32_t hash) {
MutexLock l(&mutex_);
// 借助哈希表快速定位 entry
LRUHandle* e = table_.Lookup(key, hash);
if (e != nullptr) {
// 如果找到,增加 entry 的引用计数
Ref(e);
}
return reinterpret_cast<Cache::Handle*>(e);
}
void LRUCache::Ref(LRUHandle* e) {
if (e->refs == 1 && e->in_cache) {
// 如果当前 entry 在 lru 链表中,需要将其移动到 in_use 链表,不需要等待淘汰
LRU_Remove(e);
// 添加到链表头部
LRU_Append(&in_use_, e);
}
e->refs++;
}
Release
void LRUCache::Release(Cache::Handle* handle) {
MutexLock l(&mutex_);
// 对 entry 解引用
Unref(reinterpret_cast<LRUHandle*>(handle));
}
void LRUCache::Unref(LRUHandle* e) {
assert(e->refs > 0);
// 引用计数减少
e->refs--;
if (e->refs == 0) {
// 当引用计数为 0 时进行清理
assert(!e->in_cache);
(*e->deleter)(e->key(), e->value);
free(e);
} else if (e->in_cache && e->refs == 1) {
// 当引用计数为 1 时移动到 lru 链表等待淘汰
LRU_Remove(e);
// 添加到链表头部
LRU_Append(&lru_, e);
}
}
Insert
Cache::Handle* LRUCache::Insert(const Slice& key, uint32_t hash, void* value,
size_t charge,
void (*deleter)(const Slice& key,
void* value)) {
MutexLock l(&mutex_);
LRUHandle* e =
reinterpret_cast<LRUHandle*>(malloc(sizeof(LRUHandle) - 1 + key.size()));
e->value = value;
e->deleter = deleter;
e->charge = charge;
e->key_length = key.size();
e->hash = hash;
e->in_cache = false;
// 返回 handle,引用计数加一
e->refs = 1;
std::memcpy(e->key_data, key.data(), key.size());
if (capacity_ > 0) {
// 加入缓存,引用计数加一
e->refs++;
e->in_cache = true;
LRU_Append(&in_use_, e);
usage_ += charge;
FinishErase(table_.Insert(e));
} else {
// 不缓存(支持 capacity==0,这表示关闭缓存
// next 会影响 `key()` 中的断言,因此必须对其进行初始化
e->next = nullptr;
}
while (usage_ > capacity_ && lru_.next != &lru_) {
// entry 的总消耗大于缓存的容量
LRUHandle* old = lru_.next;
assert(old->refs == 1);
bool erased = FinishErase(table_.Remove(old->key(), old->hash));
if (!erased) {
// 在编译 NDEBUG 时避免使用未使用的变量
assert(erased);
}
}
return reinterpret_cast<Cache::Handle*>(e);
}
Erase
bool LRUCache::FinishErase(LRUHandle* e) {
if (e != nullptr) {
assert(e->in_cache);
// 从 in_use 链表中删除
LRU_Remove(e);
e->in_cache = false;
usage_ -= e->charge;
// 解引用
Unref(e);
}
return e != nullptr;
}
void LRUCache::Erase(const Slice& key, uint32_t hash) {
MutexLock l(&mutex_);
FinishErase(table_.Remove(key, hash));
}
Prune
void LRUCache::Prune() {
MutexLock l(&mutex_);
// 清空 lru 链表
while (lru_.next != &lru_) {
LRUHandle* e = lru_.next;
assert(e->refs == 1);
bool erased = FinishErase(table_.Remove(e->key(), e->hash));
if (!erased) {
// 在编译 NDEBUG 时避免使用未使用的变量
assert(erased);
}
}
}
entry 的引用计数
LRUCache
中的 entry 可以分为四个状态:
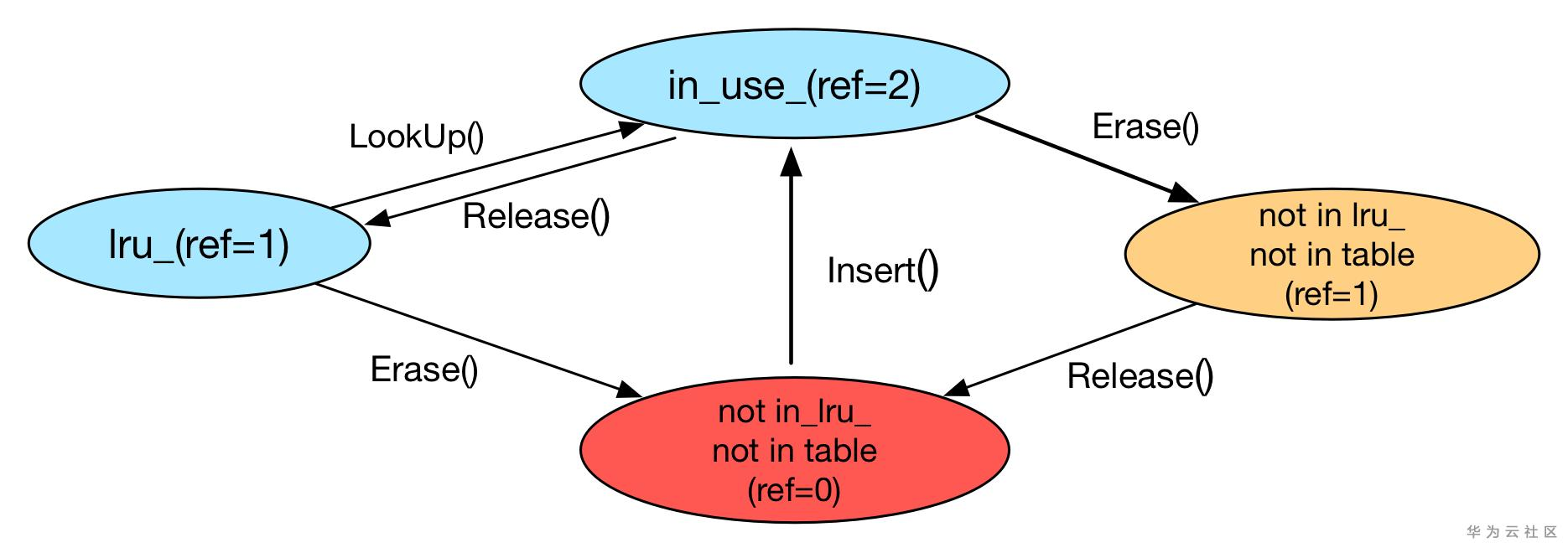
- in use (ref=2):
- 该 entry 在
table
中,并且在in_use
链表中;由于该 entry 既被外部 handle 引用,也被in_use
链表使用,因此有 ref=2; Insert
函数创建一个新的 entry 保存相应的 key-value 数据,并加入in_use
链表的表头,然后返回对应的 handle 到外部,并将 ref 设置为 2;
- 该 entry 在
- in lru (ref=1):
- 该 entry 在
table
中,并且在lru
链表中;由于该 entry 只被lru
链表使用,因此有 ref=1; Lookup
函数和Release
函数成对出现。Lookup
查找 key 对应的 entry,并返回 entry 对应的 handle 给外部,增加一个引用, ref 加 1;Release
函数则相反,释放 handle,减少一个引用,ref 减 1;
- 该 entry 在
- not in lru, not in table (ref=1):
- 该 entry 不在链表中也不在
table
中,但是仍然被外部 handle 引用且 handle 未释放,因此 ref=1; Erase
函数将 entry 从table
和in_use
中删除,减少一个引用,ref 减 1,但是若此 entry 还被外部 handle 引用,不能直接释放 entry 占用的内存,必须等外部使用者调用Release
函数之后才能释放内存;
- 该 entry 不在链表中也不在
- not in lru, not in table (ref=0):该 entry 不在链表中也不在
table
中,也不被外部使用,因此 ref=0;
ShardedLRUCache 类
ShardedLRUCache
是 LevelDB 对外暴露的 LRUCache。
static const int kNumShardBits = 4;
static const int kNumShards = 1 << kNumShardBits;
class ShardedLRUCache : public Cache {
private:
LRUCache shard_[kNumShards];
port::Mutex id_mutex_;
uint64_t last_id_;
public:
explicit ShardedLRUCache(size_t capacity) : last_id_(0) {
const size_t per_shard = (capacity + (kNumShards - 1)) / kNumShards;
for (int s = 0; s < kNumShards; s++) {
shard_[s].SetCapacity(per_shard);
}
}
}
ShardedLRUCache
中定义了 16 个 LRUCache
,每次进行插入或者查找时,使用哈希值的最高四位判断当前应该在哪个 LRUCache
中查找,然后在找到的 LRUCache
中进行相应的操作。
static inline uint32_t HashSlice(const Slice& s) {
return Hash(s.data(), s.size(), 0);
}
static uint32_t Shard(uint32_t hash) {
return hash >> (32 - kNumShardBits);
}
因为 LRUCache
的查找、插入和释放均需要加锁,而这种分片的方式能够提高 LevelDB 对于缓存的并发访问,也就是从侧面降低了锁的粒度,提高访问效率。
Cache 相关接口实现
ShardedLRUCache
的接口实现非常简单,它自己只进行哈希值的计算,然后选择相应的 LRUCache
,调用相应 LRUCache
的相关接口实现。
Handle* Insert(const Slice& key, void* value, size_t charge,
void (*deleter)(const Slice& key, void* value)) override {
const uint32_t hash = HashSlice(key);
return shard_[Shard(hash)].Insert(key, hash, value, charge, deleter);
}
Handle* Lookup(const Slice& key) override {
const uint32_t hash = HashSlice(key);
return shard_[Shard(hash)].Lookup(key, hash);
}
void Release(Handle* handle) override {
LRUHandle* h = reinterpret_cast<LRUHandle*>(handle);
shard_[Shard(h->hash)].Release(handle);
}
void Erase(const Slice& key) override {
const uint32_t hash = HashSlice(key);
shard_[Shard(hash)].Erase(key, hash);
}
void* Value(Handle* handle) override {
return reinterpret_cast<LRUHandle*>(handle)->value;
}
uint64_t NewId() override {
MutexLock l(&id_mutex_);
return ++(last_id_);
}
void Prune() override {
for (int s = 0; s < kNumShards; s++) {
shard_[s].Prune();
}
}
size_t TotalCharge() const override {
size_t total = 0;
for (int s = 0; s < kNumShards; s++) {
total += shard_[s].TotalCharge();
}
return total;
}
- 点赞
- 收藏
- 关注作者
评论(0)